Paediatric Biomechanics
Characterising the form-function relationships of the musculoskeletal system in children from their first step in order to understand biomechanics in early development and in developmental diseases.
Our research
The Auckland Bioengineering Institute (ABI) has pioneered the development of anatomically-based, patient-specific models to study biomechanical principles underlying physiological processes for advanced clinical use. Computational models of the musculoskeletal system have provided new ways to study muscle and joint function during motion and elucidate effects of musculoskeletal deformities on gait dynamics.
1. The Musculoskeletal Atlas project
The Musculoskeletal Atlas Project for paediatric Conditions such as developmental hip dysplasia, cerebral palsy and slipped capital femoral epiphysis (SCFE) can lead to complex hip and knee deformities in paediatric and adolescent patients. These conditions can lead to a large spectrum of impairments leading to heterogeneous musculoskeletal deformities which make it difficult to quantify efficacy of an individual treatment or know which interventions are most effective for which child. Therefore a personalised medicine approach is often needed necessitating patient-specific assessment. To accelerate the generation of patient-specific modelling for orthopaedic research, the Auckland Bioengineering Institute has developed the Musculoskeletal Atlas Project (MAP), an open-source software platform, to generate computational models of the musculoskeletal system. We are expanding the atlas to a paediatric population and validate its capability to:
- Predict bone geometry of children with musculoskeletal deformities using routine clinical imaging.
- Generate patient-specific neuro-musculoskeletal models of common hip and knee conditions in children.
2. The Smart Splint project
The Smart Splint project for early treatment in children with Cerebral Palsy
The Smart Splint project consists of creating a workflow to deliver personalised treatment to children with Cerebral Palsy based on their form and functional assessment (Figure 1).The first step in this workflow is to accurately capture the bone morphology and lower limb geometry using a population model (MAP) and 3D scanner technology. The second step is to capture patient-specific gait data to input into a computational model of the patient, which combineswearable sensors with machine learning algorithms to improve the assessment and monitoring of patients. The final step is to use the computational model’s output to predict treatment outcomes, custom made orthoses and personalise rehabilitation exercises.
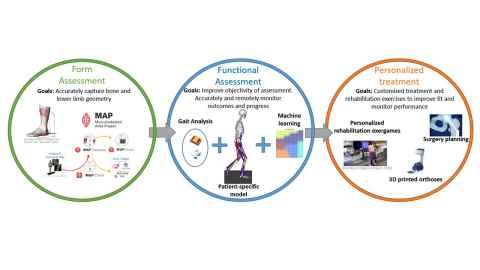
3. Muscle impairments in Cerebral Palsy
Understanding the Progression of Muscle Impairments in Cerebral Palsy:
Cerebral Palsy is the leading cause of movement disability in children. Cerebral palsy begins with a neural impairment, but there are manifest effects in the musculoskeletal system itself. Paradoxically, while the neural insult is nonprogressive, the musculoskeletal effects worsen over time. Understanding the nature of this progressive worsening is the goal of our group’s work. We use imaging and computational modeling to probe the process of CP progression in the muscles.
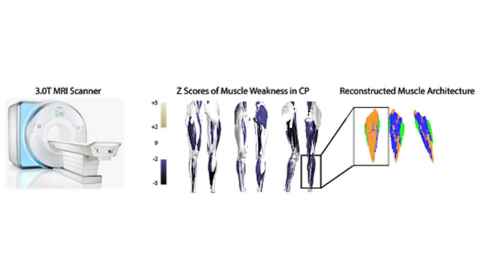
In order to understand impaired muscle growth in children with cerebral palsy, we first need to understand the process of muscle growth in typical adolescents, and apply that understanding to cerebral palsy. Working closely with collaborators at the medical school and Liggins Institute in Auckland, we have developed computational models of the process of muscle growth at the micro- and macroscopic levels. We are using novel MRI approaches to provide foundational data and validate these models.
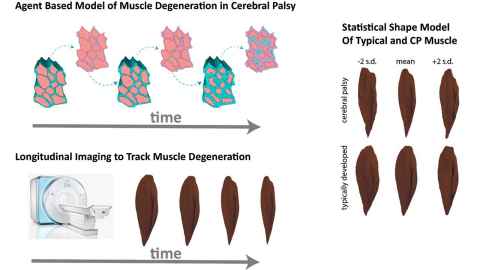
Members
Julie Choisne (1 and 2)
Geoff Handsfield (3)
Students
Funding partners
- HRC
- MedTech CoRE
- NSC-Science for Technological Innovation
- The Friedlander foundation and the Robertson Foundation (Aotearoa Fellowship)